The growing importance of medical injection molding in healthcare technology has revolutionized the production of high-precision medical devices. Medical atomization mask injection molding processing plays a crucial role in manufacturing essential components for respiratory treatments, ensuring both effectiveness and patient safety. This process allows for the creation of intricate, precise designs that meet stringent medical standards, such as biocompatibility and sterilization. Companies like FUJIU Medical Injection Mold have made significant contributions to the field, leveraging their expertise in medical-grade injection molding. By utilizing advanced molding techniques, FUJIU Medical Injection Mold and other industry leaders are helping to improve the quality, cost-efficiency, and scalability of medical atomization masks, ultimately enhancing patient care worldwide.
Low-Volume to High-Volume Production Capabilities
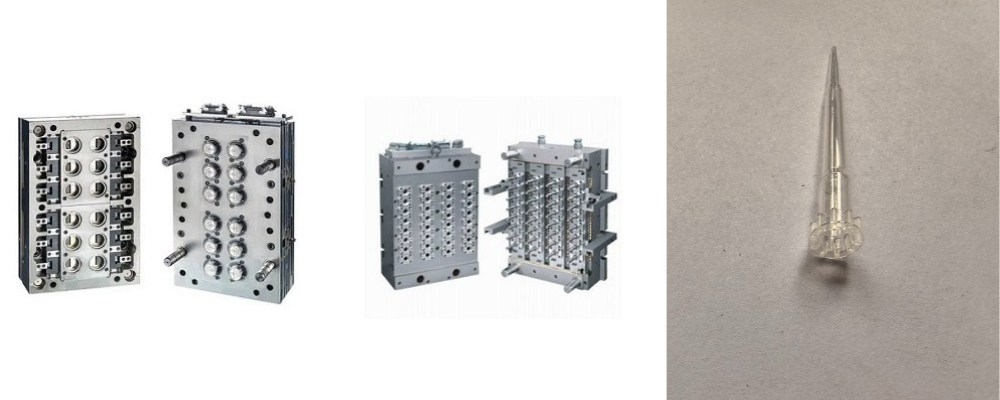
Attributes | Details |
---|---|
Place of Origin | Dongguan, Guangdong, China |
Brand Name | LY Medical Injection Mold |
Product Name | Injection Molding of Medical Atomization Masks |
Process | Injection Molding Plastic Parts |
Micro-machining or Not | Non-micro-machining |
Material | Plastic |
Tolerance | 0.01-0.05mm |
OEM/ODM | Yes |
Drawing Format | 2D/(PDF/CAD)3D(IGES/STEP) |
Logo | Customized Logo Accepted |
Product Usage | Medical Use |
Certificate | ISO13485 and ISO9001 |
Surface Treatment | Mirror Polishing |
Mold Life | 250000-300000 Shots |
Features | High Precision Injection Molded Parts |
What Is Medical Atomization Mask Injection Molding Processing
Benefits of Injection Molding for Medical Atomization Masks
Medical atomization mask injection molding processing has become a pivotal part of the manufacturing landscape for healthcare technology. This advanced method not only ensures precision and accuracy but also supports high-quality, large-scale production while meeting stringent medical standards. Here are the primary benefits of this innovative approach:
Precision and Accuracy
Injection molding for medical atomization masks enables the creation of highly intricate designs, which are essential for atomization components. The precision achieved through this process allows for the production of masks with detailed internal channels and structures that optimize airflow and medication delivery. This level of accuracy ensures that atomization devices can efficiently disperse medication, providing reliable treatment outcomes for patients.
Hygiene Compliance
One of the significant advantages of medical atomization mask injection molding processing is its ability to support cleanroom manufacturing. This process utilizes high standards of sterilization to meet regulatory requirements, such as those set by the FDA. The components produced through this method are made in environments free of contaminants, ensuring maximum hygiene and safety. The cleanroom setting also allows for meticulous quality control, making injection-molded atomization masks particularly well-suited for sensitive medical applications.
Cost-Efficiency
Another key benefit of medical atomization mask injection molding processing is its cost-efficiency. The use of advanced injection molding techniques reduces material waste, as the precise nature of the molds allows for efficient use of raw materials. This not only minimizes costs but also contributes to environmental sustainability. The automation of the medical injection molding process further reduces labor and operational expenses, making it a cost-effective solution for the high-volume production needed in the medical sector.
High Volume Reproducibility
Injection molding for medical atomization masks is capable of producing high volumes of identical components with consistent quality. This level of reproducibility is essential for meeting the demands of large-scale medical device production. By using specialized molds and materials, manufacturers can efficiently scale up production while maintaining the necessary precision and integrity of each component. This ensures that atomization masks can be produced rapidly and affordably, meeting the needs of patients and healthcare providers alike.
Medical atomization mask injection molding processing continues to advance through continuous innovations in material science and manufacturing techniques, providing the healthcare industry with high-quality, efficient, and reliable solutions. This method not only benefits from precision and efficiency but also supports regulatory compliance and sustainability, making it an indispensable process in modern medical device plastic manufacturing.
Materials Used in Medical Atomization Mask Injection Molding Processing
Medical atomization mask injection molding involves the use of specialized materials that are selected based on their performance, safety, and compatibility with medical standards. The materials used in the injection molding process of medical atomization masks must meet stringent requirements, including biocompatibility, durability, and the ability to maintain structural integrity while being comfortable for patients. Below is a detailed description of the materials commonly used in the medical atomization mask injection molding processing:
Polypropylene (PP)
- Properties: Polypropylene is one of the most commonly used materials in the production of medical atomization masks. It is a thermoplastic polymer known for its excellent chemical resistance, durability, and relatively low cost.
- Advantages: PP is non-toxic, has good fatigue resistance, and is lightweight, making it ideal for disposable medical products like masks. Additionally, it has low moisture absorption and is easy to process.
- Applications: It is primarily used for components of the mask structure, including the body and components that need to withstand frequent sterilization processes.
Polycarbonate (PC)
- Properties: Polycarbonate is another widely used plastic in medical applications. It is known for its excellent impact resistance, high transparency, and ability to withstand high temperatures.
- Advantages: PC is biocompatible, meaning it is safe for use in medical applications where direct contact with patients is involved. It is also easy to mold, which is beneficial for creating the intricate designs required for atomization masks.
- Applications: Polycarbonate is often used for parts of the mask that require higher strength or transparency, such as the face shield or the transparent parts of the mask.
Medical-Grade Silicone (MSR or LSR)
- Properties: Liquid silicone rubber (LSR) and medical-grade silicone rubber (MSR) are materials commonly used in medical device manufacturing, including atomization masks. These materials are flexible, durable, and biocompatible.
- Advantages: Medical-grade silicones are known for their exceptional comfort, making them ideal for the parts of the mask that come into direct contact with the patient’s skin, such as the sealing edges or the straps. They are also resistant to degradation over time, and they can withstand sterilization without losing their properties.
- Applications: Silicone is commonly used in making the soft sealing portions of atomization masks to ensure a secure and comfortable fit.
Acrylonitrile Butadiene Styrene (ABS)
- Properties: ABS is a versatile thermoplastic material known for its toughness, impact resistance, and ease of molding. It also offers a good balance of rigidity and flexibility.
- Advantages: ABS is relatively cost-effective, making it an attractive choice for medical injection molding. It is also resistant to certain chemicals and has good dimensional stability, which is crucial for the performance of atomization masks.
- Applications: ABS is used in the structure of the atomization mask, including non-contact areas where high rigidity and impact resistance are required.
Polyethylene (PE)
- Properties: Polyethylene, especially high-density polyethylene (HDPE), is used in medical device manufacturing due to its biocompatibility, low cost, and ease of processing.
- Advantages: PE has low friction, high chemical resistance, and is lightweight, which are beneficial for creating masks that are both comfortable and easy to clean.
- Applications: PE is often used in the mask’s components that don’t require high strength but still need to be resistant to moisture and chemicals.
Polyvinyl Chloride (PVC)
- Properties: PVC is a widely used thermoplastic polymer in medical applications. It can be manufactured in a rigid or flexible form, depending on the needs of the application.
- Advantages: PVC is durable, cost-effective, and can be easily molded into complex shapes. Flexible PVC, in particular, is used for its soft and pliable properties, which are beneficial for comfort and secure fit.
- Applications: Flexible PVC is used for parts like tubing and flexible components of the mask, such as the nose or mouth areas.
Thermoplastic Elastomers (TPE)
- Properties: TPEs combine the properties of both elastomers (rubber-like flexibility) and thermoplastics. They are used where flexibility, elasticity, and a soft-touch feel are required.
- Advantages: TPEs are biocompatible, flexible, and resistant to UV degradation, making them ideal for medical applications. They also offer a high degree of moldability, which is essential for creating comfortable components of atomization masks.
- Applications: TPE is often used in the sealing elements of masks to ensure a snug fit while providing a soft and comfortable feel on the skin.
Polyamide (Nylon)
- Properties: Nylon is a strong, durable, and wear-resistant material commonly used in medical devices. It offers excellent mechanical properties and dimensional stability.
- Advantages: Nylon has good chemical resistance and is capable of withstanding high temperatures. Its strength and durability make it suitable for parts of the atomization mask that require high structural integrity.
- Applications: Nylon is often used in the mask frame or structural elements that need to support the mask’s other parts.
Polystyrene (PS)
- Properties: Polystyrene is a transparent thermoplastic that can be rigid or foam-based. It is often used for medical components that require clear visibility and lightness.
- Advantages: It is easy to mold and process, and it provides clarity for transparent components. It also offers moderate impact resistance.
- Applications: It is typically used in the transparent parts of the atomization mask, such as the face shield or mouthpiece.
Considerations for Material Selection
- Biocompatibility: The materials used must not cause adverse reactions when in contact with human skin or respiratory systems. This is crucial for medical devices.
- Sterilization: The chosen materials should be capable of withstanding sterilization processes such as autoclaving, UV treatment, or gamma radiation.
- Durability and Performance: The materials must provide the required mechanical strength, flexibility, and chemical resistance to ensure the mask’s longevity and functionality.
- Comfort: Materials that contact the skin should be non-irritating, flexible, and soft to provide comfort during extended wear.
The selection of materials for medical atomization masks is critical to ensuring both patient safety and the effective performance of the device. The combination of materials like polypropylene, polycarbonate, silicone, and thermoplastic elastomers ensures that the mask can provide both comfort and durability while meeting medical standards.
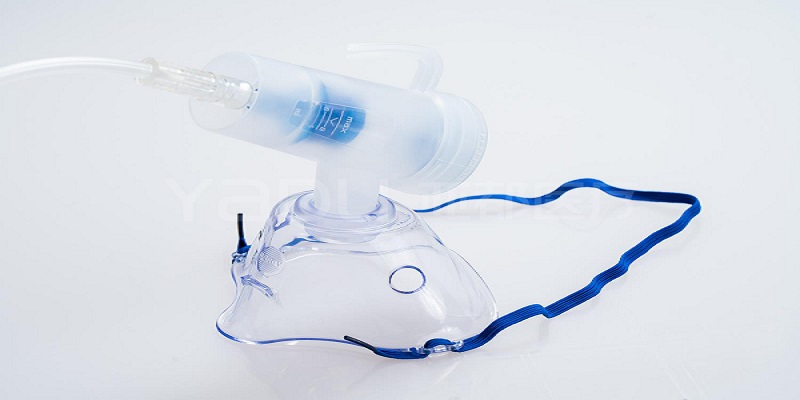
Choice Factors for Medical Atomization Mask Injection Molding Processing Materials
When selecting materials for medical atomization mask injection molding processing, several factors need to be considered to ensure the product’s safety, performance, and compliance with regulatory standards. The following are the key choice factors that play a critical role in the material selection process:
Biocompatibility
- Explanation: Biocompatibility refers to the ability of a material to interact safely with the human body without causing adverse reactions. In the case of medical atomization masks, materials must be non-toxic, non-irritating, and not provoke allergic reactions when in contact with the skin or respiratory systems.
- Why It Matters: Since atomization masks are used in close contact with the patient’s face and respiratory system, materials must meet stringent biocompatibility standards to avoid harmful effects such as skin irritation, sensitization, or respiratory problems.
- Example: Materials like medical-grade silicone, polypropylene, and polycarbonate are often chosen because they have established safety profiles in medical devices.
Sterilization Compatibility
- Explanation: The materials used must withstand various sterilization methods such as autoclaving (steam sterilization), gamma radiation, or chemical sterilization without degrading or losing their properties.
- Why It Matters: Atomization masks are typically designed for repeated use, and ensuring they can be safely sterilized is crucial for patient safety. Incompatible materials might deform, lose functionality, or leach harmful substances during sterilization.
- Example: Polypropylene and polycarbonate are good options because they can endure high-temperature sterilization processes without significant degradation.
Durability and Mechanical Properties
- Explanation: Medical devices like atomization masks need to maintain their structural integrity over time. The materials should be resistant to wear, tear, and physical deformation, ensuring they retain their shape and function during prolonged use.
- Why It Matters: Atomization masks should not crack, break, or lose shape, especially when subjected to repeated handling, cleaning, and sterilization. Durability ensures that the mask will perform as expected throughout its lifecycle.
- Example: Materials like polycarbonate and nylon offer high strength and impact resistance, making them ideal for structural components of the mask.
Comfort and Fit
- Explanation: Since atomization masks are worn over the face, comfort and fit are key factors. Materials must be flexible and soft where direct contact with the skin occurs, such as at the edges of the mask or the straps.
- Why It Matters: A mask that is uncomfortable or ill-fitting can cause irritation or even lead to user non-compliance, reducing its effectiveness. Comfortable materials also enhance the patient’s ability to wear the mask for extended periods, which is essential in medical treatments such as nebulization.
- Example: Soft, flexible materials like silicone and thermoplastic elastomers (TPE) are commonly used in areas that need to provide a soft, skin-friendly seal.
Transparency
- Explanation: Many atomization masks have transparent components (e.g., face shields) to allow healthcare professionals to visually monitor the patient’s condition, especially during procedures like nebulization.
- Why It Matters: Transparency is vital for visual inspection of the patient’s breathing and overall comfort during the treatment. Materials must provide adequate clarity without compromising strength.
- Example: Polycarbonate and polystyrene are often chosen for transparent components because they offer high clarity and are impact-resistant.
Chemical Resistance
- Explanation: Materials must be resistant to the chemicals that are used in the mask’s environment, including medications, cleaning agents, and sterilizing solutions.
- Why It Matters: Exposure to chemicals during use or cleaning can lead to material degradation, discoloration, or loss of mechanical properties. Masks that are chemically resistant are more durable and maintain their functionality over time.
- Example: Polypropylene and polycarbonate are known for their resistance to common cleaning chemicals, which helps in maintaining the mask’s integrity after multiple cleanings.
Ease of Manufacturing
- Explanation: The material should be easy to process using injection molding techniques. It should flow well through the molds and allow for the creation of intricate shapes or designs that are often required in medical devices like atomization masks.
- Why It Matters: Materials that are difficult to mold or process can result in high production costs, longer cycle times, and potentially lower-quality products. Efficient manufacturing ensures that the final product meets quality standards while maintaining cost-effectiveness.
- Example: Thermoplastics like polypropylene, polyethylene, and ABS are widely used in injection molding because of their ease of processing and cost-effectiveness.
Weight
- Explanation: The weight of the atomization mask affects the comfort and ease of use. A lightweight material makes the mask more comfortable for patients, especially when worn for extended periods.
- Why It Matters: A heavy mask may cause discomfort, and long-term use may lead to user fatigue, which could impact the effectiveness of treatment.
- Example: Polypropylene and polyethylene are lightweight materials that help reduce the overall weight of the mask, enhancing patient comfort.
Cost-Effectiveness
- Explanation: While performance and safety are the highest priorities, the cost of the material must also be considered. The material should not significantly increase the overall cost of manufacturing, as medical devices often require mass production.
- Why It Matters: Medical products must balance quality with cost to make them accessible to patients and healthcare providers while maintaining profitability for manufacturers.
- Example: Materials like polypropylene and polystyrene are relatively low-cost, making them attractive choices for mass production.
The choice of materials for medical atomization mask injection molding processing must be made with careful consideration of factors like biocompatibility, sterilization compatibility, durability, comfort, and cost-effectiveness. The final decision depends on the specific requirements of the mask, including its intended use, patient comfort, regulatory standards, and the manufacturing capabilities of the producer. By balancing these factors, manufacturers can produce high-quality, safe, and effective atomization masks that meet both medical standards and patient needs.
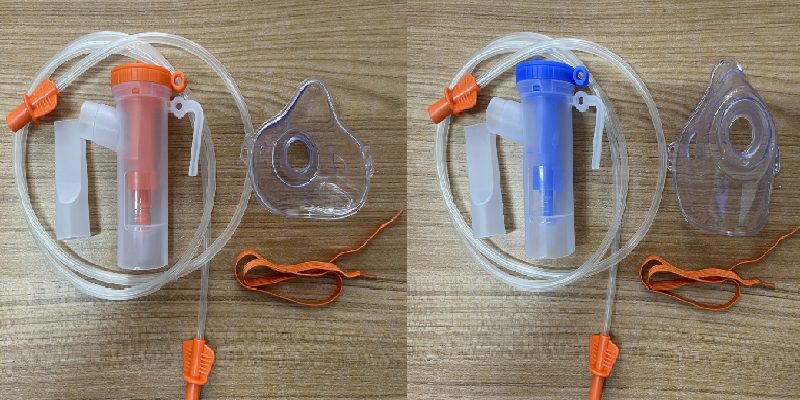
Key Injection Molding Techniques for Atomization Masks
Medical atomization mask injection molding processing relies on several advanced injection molding techniques to produce high-quality, efficient medical devices. These techniques are tailored to meet the unique needs of atomization masks, which require precision, comfort, and reliability. Below are the key injection molding methods used in the production of medical atomization masks:
Thin Wall Molding
Thin wall molding is a critical technique in customization wholesale injection molding of medical atomization mask, particularly for ensuring lightweight and comfortable designs. This method allows manufacturers to produce atomization masks with extremely thin walls, typically under 1mm in thickness. The thin walls not only reduce the overall weight of the mask, making it more comfortable for prolonged use, but also improve the mask’s flexibility and fit. This is crucial for ensuring that the mask securely adheres to the patient’s face, creating a better seal and enhancing the efficiency of atomization therapy. Additionally, thin wall molding helps reduce material usage, making the production process more cost-effective and environmentally friendly while maintaining high performance.
Liquid Silicone Injection Molding
Liquid medical silicone injection molding (LSR) is another key technique used in medical atomization mask injection molding processing. LSR is particularly well-suited for producing soft, flexible components required for medical atomization masks. Silicone materials are biocompatible, resistant to chemicals, and have excellent temperature stability, making them ideal for applications where patient safety and comfort are a priority. This technique allows for the production of flexible, durable masks that can withstand repeated sterilization cycles without degrading. Liquid silicone molding also ensures smooth, consistent parts that enhance the overall patient experience by providing a secure and comfortable fit during respiratory treatments.
Gas-Assisted Injection Molding
Gas-assisted injection molding is a specialized technique used in medical atomization mask injection molding processing to improve the structural integrity and appearance of complex parts. This method involves injecting a gas, usually nitrogen, into the mold cavity during the injection process. The gas creates pressure that helps the molten material fill the mold more uniformly, reducing the likelihood of defects such as sink marks or warping. This results in atomization masks with improved structural integrity, ensuring that each mask performs reliably throughout its lifecycle. Additionally, gas-assisted molding allows for the creation of more intricate designs, such as hollow sections or complex internal channels, without compromising the quality or functionality of the mask.
These advanced injection molding techniques play a crucial role in producing high-quality, reliable medical atomization masks. Each technique offers unique advantages that address the specific needs of atomization therapy, including lightweight designs, flexibility, structural integrity, and patient comfort. By utilizing these techniques, manufacturers ensure that medical atomization mask injection molding processing can meet the high standards required for medical applications, all while improving production efficiency and scalability.
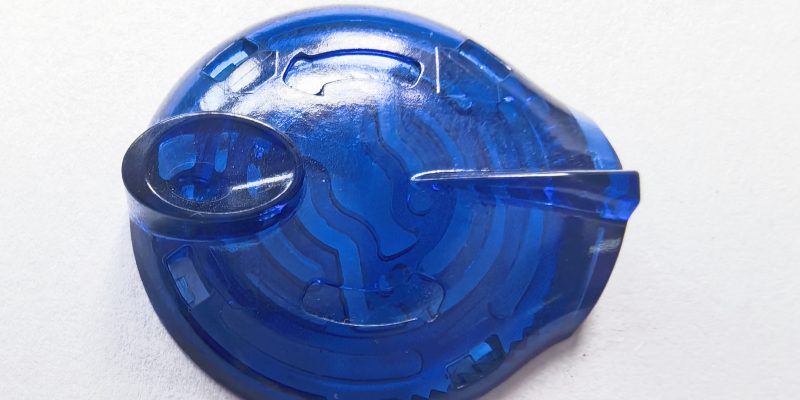
The Manufacturing Process Medical Atomization Mask Injection Molding Processing
The manufacturing process of medical atomization masks using injection molding involves several key steps that ensure high precision, consistency, and quality for the final product. Below is a detailed overview of the medical atomization mask injection molding processing:
Material Selection
- Description: The first step in the manufacturing process is selecting the appropriate material for the atomization mask. The material needs to meet various requirements, such as biocompatibility, chemical resistance, flexibility, durability, and ease of sterilization.
- Common Materials: Medical-grade polypropylene (PP), polycarbonate (PC), silicone, thermoplastic elastomers (TPE), and polyethylene (PE) are commonly used in the injection molding of atomization masks.
Injection Molding Machine Setup
- Description: The injection molding process begins with setting up the injection molding machine. This includes selecting the correct mold for the atomization mask components and ensuring the machine is set to the optimal temperature, pressure, and injection speed based on the material being used.
- Components of the Injection Molding Machine:
- Hopper: Holds the raw plastic material in pellet or granule form.
- Barrel: Melts the material and prepares it for injection.
- Injection Unit: Injects the molten plastic into the mold cavity.
- Mold: A metal tool that shapes the final part of the mask. It consists of two halves: the core and cavity, which form the mold shape when closed.
Injection of Material
- Description: Once the material is melted and prepared, the injection molding machine injects it into the mold under high pressure. The molten plastic flows into the mold cavity, filling it completely and capturing all the fine details of the mask design.
- Process Details:
- The machine forces the molten material into the mold cavity at high pressure to ensure it fills the mold completely.
- The injection speed and pressure must be carefully controlled to avoid defects such as air bubbles, incomplete filling, or warping.
Cooling and Solidification
- Description: After the mold cavity is filled, the material needs to cool and solidify. This cooling process is crucial as it helps the plastic take the correct shape and solidify properly without deforming.
- Process Details:
- The mold is often equipped with cooling channels to circulate coolant (usually water) around the mold to lower the temperature.
- The cooling time depends on the type of material used, the thickness of the component, and the size of the mold. Materials like polypropylene and polycarbonate typically cool faster than silicone or thermoplastic elastomers.
Mold Opening and Part Ejection
- Description: Once the plastic has cooled and solidified, the mold opens, and the atomization mask is ejected. Ejection mechanisms, such as ejector pins or air blasts, are used to remove the part from the mold without causing damage.
- Process Details:
- The mold opens, and ejector pins push the solidified part out of the mold cavity.
- Sometimes, a robotic arm or automated handling system may be used to pick up the mask for further processing.
Trimming and Finishing
- Description: After ejection, the mask may have excess material, such as sprues (leftover material from the injection point) or flash (excess plastic that leaks from the mold cavity). These need to be trimmed away to ensure the mask has the correct shape and meets specifications.
- Process Details:
- Manual Trimming: Some excess material is trimmed manually, especially if it is a small amount.
- Automated Trimming: For larger batches, an automated trimming system may be used to remove sprues, runners, and flash.
- Finishing: In some cases, additional finishing operations such as surface polishing, cleaning, or coating may be performed to improve the appearance or functionality of the mask.
Assembly (If Applicable)
- Description: In some cases, the atomization mask may consist of multiple components that need to be assembled. This could include adding parts like valves, filters, or straps.
- Process Details:
- The individual components of the mask are assembled either manually or using automation. This could involve ultrasonic welding, snap-fit assembly, or other joining methods to ensure secure attachment of different parts.
- If the mask has a flexible silicone seal, it may be attached during the assembly stage.
Quality Control and Inspection
- Description: Quality control is a critical part of the manufacturing process to ensure the atomization masks meet the required medical standards and specifications. Various inspection techniques are used to detect defects, ensure proper fit, and verify that the masks meet regulatory requirements.
- Inspection Methods:
- Visual Inspection: Check for any visible defects, such as cracks, scratches, or discoloration.
- Dimensional Inspection: Ensure that the mask’s dimensions are within specified tolerances using tools such as calipers, micrometers, or automated vision systems.
- Leak Testing: Ensure that the mask seals properly, especially around the edges, to avoid air or fluid leakage.
- Biocompatibility Testing: The material undergoes testing to ensure it meets biocompatibility standards, especially for masks used in direct contact with patients.
Packaging
- Description: After passing quality control, the atomization masks are cleaned (if needed), packaged, and prepared for shipping. Medical devices must be carefully packaged to maintain sterility and prevent contamination.
- Packaging Methods:
- Sterile Packaging: The masks are often packaged in sterile pouches or blister packs to ensure that they remain sterile until they are used.
- Labeling: Proper labeling is done to indicate the product’s specifications, sterilization status, and expiration date, if applicable.
- Packaging for Distribution: The packaged atomization masks are then placed in boxes for distribution to healthcare providers.
Final Sterilization (If Applicable)
- Description: In some cases, the atomization masks may undergo final sterilization after manufacturing, especially if they are reusable or intended for medical use. Sterilization methods include autoclaving, UV sterilization, or gamma radiation.
- Process Details:
- Autoclaving: Uses steam under pressure to sterilize the masks.
- Gamma Radiation: Often used for packaging and sterilizing disposable masks.
- UV Sterilization: For specific components, UV light is used to kill any bacteria or viruses on the surface.
The injection molding process for manufacturing medical atomization masks involves several key stages, including material selection, injection molding, cooling, ejection, trimming, quality control, and final packaging. The entire process is designed to meet stringent medical standards and ensure that the final product is safe, functional, and comfortable for patients. Proper attention to detail in each step of the process helps ensure that the atomization masks provide effective and reliable performance during medical treatments.
Medical Atomization Mask Injection Molding Processing Factory – FUJIU Medical Injection Mold
At FUJIU Medical Injection Mold, all stages in the product development process occur under one roof, including product design, precision tooling, manufacturing, and fulfillment.
By involving our in-house tooling team from the start, we’ll help you develop an elegant product design that prioritizes use, manufacturability, and material conservation. Some of the many benefits of our one-roof approach include:
- We understand the unique properties of different types of silicone and thermoplastic. With decades of medical, industrial, and consumer product design experience, our unique team of OEM engineers will work with you to create the best, most cost-effective version of your product.
- We take manufacturability—the ease at which a product can be injection molded at scale—into account from Day 1. From the drawing board, our production team is involved to help us optimize your product design for production.
- Unlike other factories that send your mold out for initial precision tooling and any future adjustments, FUJIU Medical Injection Mold has an in-house tool shop. This means that potential mold adjustments are more affordable and have higher turnaround times.
FUJIU Medical Injection Mold is pleased to offer expert medical device manufacturing for class I and class II devices. In addition to ISO 13485:2016 certification, FUJIU Medical Injection Mold is the manufacturer on record for many FDA-registered products.
Expertise in Medical-Grade Injection Molding
At FUJIU Medical Injection Mold, we bring over 20 years of excellence as a third-generation, employee-owned company. Every team member is a stakeholder, fueling our passion for innovation, quality, and collaboration. We specialize in OEM contract manufacturing for the medical device industry, producing high-precision plastic consumables for equipment and diagnostic kits. From initial design to production, we partner with clients using advanced injection molding technologies. Equipped with Class 8 clean rooms and adhering to ISO 13485, FDA, and global standards, we ensure the highest quality control. Our unique blend of family values and employee empowerment sets us apart, guaranteeing a sustainable future for our team and clients worldwide.
Our Mission: Precision, Integrity, and Innovation
At FUJIU Medical Injection Mold, our mission is to be more than just a manufacturer—we strive to be a trusted partner in your success. Specializing in custom precision medical molds and injection-molded plastic products, we are dedicated to delivering exceptional value and unparalleled service to the medical industry.
We believe that mutual success is built on strong partnerships. By fostering open communication and collaboration, we work closely with our clients to understand their unique needs and challenges. Our team is committed to upholding the highest ethical standards, ensuring transparency and integrity in all our dealings. Continuous improvement is at the heart of our philosophy, driving us to refine our processes and enhance our capabilities at every stage of our business operations.
Challenges in Medical Atomization Mask Injection Molding Processing
The production of medical atomization masks through injection molding presents several challenges that require careful consideration and expertise. These challenges must be addressed to ensure the final product meets the highest safety, performance, and environmental standards. Below are the key challenges in medical atomization mask injection molding processing:
Adhering to Stringent Medical Regulations
One of the most significant challenges in medical atomization mask injection molding processing is adhering to the stringent medical regulations set by governing bodies such as the FDA and other regulatory agencies. These regulations are designed to ensure that medical devices, including atomization masks, are safe for patient use and perform as expected. Manufacturers must comply with good manufacturing practices (GMP), maintain traceability of the materials used, and follow detailed testing procedures to ensure that each mask is free from contaminants and defects. This rigorous process requires constant monitoring, documentation, and quality control throughout the production cycle, making it one of the more complex aspects of medical injection molding.
Balancing Cost Efficiency with Precision
Another challenge in medical atomization mask injection molding processing is balancing cost efficiency with the high precision required for medical device manufacturing. While the wholesale injection molding of medical atomization mask offers cost advantages through its ability to produce large quantities of parts quickly and efficiently, the process must still meet the precise specifications necessary for medical applications. Atomization masks, in particular, require intricate designs, smooth surfaces, and perfect functionality. Manufacturers must optimize production processes to minimize material waste, reduce cycle times, and keep production costs low, all while maintaining the tight tolerances and high-quality standards needed for safe medical use. Achieving this balance is crucial in making medical atomization masks both affordable for healthcare providers and reliable for patient care.
Incorporating Sustainable Practices into Production
Sustainability has become an increasingly important concern in the manufacturing of medical devices, including in medical atomization mask injection molding processing. As the demand for medical products grows, so does the need for manufacturers to adopt more sustainable practices. This includes reducing energy consumption, minimizing waste, and using eco-friendly materials when possible. Additionally, manufacturers are exploring ways to recycle or repurpose plastic waste and improve the overall lifecycle of products. However, incorporating sustainability into medical device production presents challenges, as the materials used must still meet high standards of quality and safety. Finding the right balance between sustainability and performance is an ongoing challenge for manufacturers in the medical injection molding industry.
Future Trends in Medical Atomization Mask Injection Molding Processing
As the healthcare industry continues to evolve, the production of medical atomization masks through injection molding is set to undergo significant changes. These changes are driven by innovations in technology, materials, and processes. The future of medical atomization mask injection molding processing will likely feature the integration of advanced techniques that improve precision, sustainability, and scalability. Below are some of the key future trends in this field:
Integration of 3D Printing for Mold Prototyping
One of the most promising trends in medical atomization mask injection molding processing is the integration of 3D printing for mold prototyping. Traditional injection molding requires precise medical mold designs that are time-consuming and costly to produce. However, with 3D printing, manufacturers can quickly create prototype molds for atomization masks, allowing for rapid testing and design iterations. This integration of 3D printing into the prototyping process reduces the time required to develop new products, accelerates innovation, and minimizes the risk of costly errors in the final molding process. As 3D printing technologies continue to improve, it is expected that they will play an even larger role in medical device manufacturing, particularly for custom or small-batch production of atomization masks.
Development of Bio-Based Plastics for Increased Sustainability
Another key trend in medical atomization mask injection molding processing is the development of bio-based plastics. As sustainability becomes a more pressing concern, manufacturers are increasingly looking for ways to reduce their reliance on petroleum-based plastics. Bio-based plastics, made from renewable resources such as plant-based materials, offer a potential solution. These materials are biodegradable, and their production can have a lower environmental impact compared to traditional plastics. In the medical device industry, bio-based plastics must still meet the stringent safety and performance standards required for medical applications, and research is ongoing to develop materials that are both sustainable and suitable for use in medical atomization masks. This shift toward bio-based plastics will likely be a major trend in the coming years, as manufacturers strive to reduce their environmental footprint.
Enhanced Automation for Improved Scalability and Precision
The future of medical atomization mask injection molding processing will also see enhanced automation in the manufacturing process. Automation offers numerous benefits, including improved scalability, faster production cycles, and increased precision. In medical device manufacturing, where tight tolerances and high-quality standards are essential, automation can help reduce human error and ensure consistent results across large production runs. With the use of robotics, AI, and machine learning, manufacturers will be able to optimize the injection molding process for atomization masks, resulting in greater efficiency and cost-effectiveness. Additionally, enhanced automation can help address the growing demand for high-volume production while maintaining the necessary precision for medical applications.
FAQs about Medical Atomization Mask Injection Molding Processing
What is the difference between injection molding and reaction injection molding?
Injection molding and reaction injection molding (RIM) are two distinct methods used in manufacturing medical products, but they differ in the materials and processes involved.
Injection molding involves the melting of plastic pellets or granules, which are then injected into a mold under high pressure to form the desired shape. This process is used for thermoplastic materials like polypropylene, polycarbonate, and polyethylene, which can be reheated and reshaped. Injection molding is widely used in the medical industry for producing products such as syringe components, medical device housings, and disposable items like IV connectors and catheters.
Reaction injection molding (RIM), on the other hand, involves the mixing of two liquid components, typically a polyol and an isocyanate, which chemically react to form a solid polymer inside the mold. RIM is commonly used for creating parts with complex geometries and is ideal for thermosetting materials like polyurethanes, which cannot be re-melted after setting. Medical applications for RIM include production of parts for medical imaging equipment, orthopedic devices, and prosthetics.
The key difference is that injection molding uses heat to melt thermoplastic materials, while RIM uses a chemical reaction to form thermosetting plastics. This makes RIM better for producing larger, lightweight parts with complex shapes, while injection molding is often more suitable for high-volume production of smaller, simpler components. Both methods have their place in the medical industry based on the requirements of the product.
What is the injection molding process simplified?
The injection molding process is a widely used manufacturing method for producing plastic parts, and it can be simplified into several basic steps. This process is particularly relevant in the medical field for creating precise and complex medical devices, such as drug delivery devices, surgical instruments, and diagnostic tools.
Material Preparation: The raw plastic material (typically in pellet form) is loaded into a hopper. For medical applications, these materials are often biocompatible plastics like polypropylene, polyethylene, or medical-grade PVC.
Melting: The plastic pellets are heated in a barrel to a molten state, ready for injection. The material reaches a high enough temperature to become viscous, ensuring it can be injected into the mold cavity.
Injection: The molten plastic is then injected into a mold under high pressure using a plunger or screw mechanism. The mold is the negative of the desired shape, and it is tightly clamped to avoid leakage.
Cooling: Once the mold is filled, the material is cooled. As the plastic cools, it solidifies to take the form of the mold. In the medical field, precise temperature control during cooling is crucial to ensure high-quality, defect-free products.
Ejection: After the material has cooled and solidified, the mold opens, and the part is ejected. A robotic arm or mechanical ejector can be used to remove the molded piece from the mold.
Finishing: After ejection, the part might require some post-processing steps like trimming, assembly, or sterilization, depending on the medical application.
This process allows for high precision, making it suitable for manufacturing complex parts in large volumes, which is especially beneficial in the medical industry where consistency and reliability are critical.
What is the injection molding theory?
The theory of injection molding is grounded in the principles of thermodynamics and fluid mechanics, particularly as they pertain to the flow and cooling of molten plastic within a mold. In the medical device industry, understanding this theory is crucial for ensuring high-quality products that meet stringent regulatory standards.
The process starts with plastic pellets or granules, which are heated in a barrel to a molten state. This molten plastic is then injected into a mold under high pressure, where it fills the cavity. The key theory here is based on the behavior of the plastic as it is injected. Factors like temperature, pressure, and flow rate must be precisely controlled to ensure that the material fills the mold correctly and completely.
Injection molding relies heavily on the concept of viscosity, which describes the material’s resistance to flow. As the plastic enters the mold, its viscosity decreases with increased temperature and shear forces, allowing it to flow easily through intricate mold cavities. The cooling of the plastic is another key component of the theory. After injection, the molten plastic cools and solidifies, with the cooling rate directly influencing the quality of the final product.
In the context of medical applications, injection molding theory is applied to ensure parts have the necessary strength, durability, and biocompatibility. For example, for surgical tools or implants, the cooling rate and mold design must be optimized to avoid defects like warping, sink marks, or voids, which could compromise product performance or patient safety.
What is the standard injection molding process?
The standard injection molding process is a critical method for producing high-precision, high-volume parts, especially in industries like medical device manufacturing. The steps involved in the standard injection molding process are as follows:
Material Selection: For medical applications, materials like medical-grade polyethylene, polypropylene, polycarbonate, and other biocompatible plastics are chosen based on their strength, flexibility, and sterilization properties.
Mold Preparation: The mold, typically made from steel or aluminum, is designed to reflect the negative shape of the part. In the medical industry, the mold is often designed with tight tolerances to ensure the final product meets strict quality standards.
Injection: The plastic material is heated until it becomes molten and is injected into the mold cavity under high pressure. For medical devices, precise control of injection pressure and speed is essential to achieve accurate, defect-free parts.
Cooling: After the mold is filled, the plastic cools and solidifies inside the mold. Cooling channels within the mold help regulate the temperature, which is crucial in medical applications to avoid thermal degradation of the plastic or the creation of internal stresses that could affect performance.
Ejection: Once the material has cooled sufficiently, the mold opens, and the part is ejected. For medical components, this process is typically automated to ensure precision and reduce human error.
Post-processing: Depending on the part, additional steps such as trimming, quality inspections, and sterilization may be required. Medical products, in particular, often undergo rigorous testing and validation to meet regulatory requirements.
The standard injection molding process is efficient for mass production of high-precision parts, ensuring consistency and quality in the manufacturing of medical devices such as syringes, catheters, and surgical tools.
What is the process of injection molding mold change?
Changing the mold in an injection molding process is a critical step, especially in a medical manufacturing setting where precision is paramount. The process of changing the mold typically involves several steps:
Preparation: Before the mold change, the machine is powered down and cooled. The operator ensures that all the necessary tools and safety equipment are ready for the mold change. In the medical industry, this step is crucial to avoid contamination of molds used for sterile products.
Removal of the Old Mold: The existing mold is carefully removed from the machine’s platen. This involves loosening any locking mechanisms, bolts, or clamps securing the mold. The operator may use hoists or robotic arms for heavy or complex molds.
Installation of the New Mold: The new mold, which has been pre-assembled and cleaned, is placed onto the machine. Alignment pins and locking mechanisms are used to ensure that the mold is positioned correctly in the injection machine.
Connection and Adjustment: The mold is then locked into place, and the machine is adjusted for the new mold size and configuration. For medical devices, precision in mold alignment is critical to ensure the final part meets all regulatory standards.
Testing: Once the new mold is in place, the machine is tested with a few trial injections. This step is to ensure the mold is functioning correctly, with proper filling, cooling, and ejection. In medical device manufacturing, any mold change must undergo strict testing to ensure the parts meet the required standards.
Final Check and Production: After confirming that the mold works properly, production resumes. The new mold will go through the injection process, and the machine settings will be monitored closely to ensure high-quality medical parts.
Changing the mold is a delicate process that requires precision, safety, and attention to detail, particularly when manufacturing medical products that must meet strict safety and quality standards.
Is injection molding difficult?
Injection molding is not inherently difficult, but it does require precision, knowledge of materials, and an understanding of the process to produce high-quality products, especially in the medical field where stringent standards must be met. The difficulty can arise in various stages, such as mold design, material selection, and process optimization.
For medical devices, the mold must be carefully designed to ensure it meets the exact specifications and tolerances needed for each component. This can be challenging due to the complexity of medical parts, such as micro-sized components used in diagnostic equipment or delicate surgical tools. The mold needs to be built to high tolerances to avoid issues like warping, defects, or contamination, which can compromise the functionality of medical devices.
Furthermore, controlling the injection molding parameters, such as pressure, temperature, and cooling rate, is critical in the production of medical products. Any fluctuation can lead to defects, which can have significant implications in medical settings. For example, defective parts may not function as intended, leading to compromised patient safety.
Despite these challenges, injection molding is a highly efficient and reliable method for mass-producing medical components. With proper training, experience, and quality control, the process can be executed successfully, producing high volumes of precise, consistent, and reliable parts for the medical industry.
Is casting cheaper than injection molding?
Casting and injection molding are both popular manufacturing processes, but the cost comparison between the two depends on several factors, including material, complexity, volume, and part design. In the medical industry, both methods can be used for different types of products, but typically, injection molding is more cost-effective for high-volume production of small, precise parts.
Casting, which involves pouring molten material into a mold and allowing it to solidify, is generally more affordable for producing low-volume or prototype parts. It may be used for larger components like prosthetics or medical equipment housings. However, casting can have limitations in terms of part complexity, precision, and material choice, which are critical factors in the medical industry where quality and biocompatibility are paramount.
In contrast, injection molding is more expensive initially due to the cost of molds and setup. However, the efficiency of the injection molding process—especially for high-volume production—can lower per-unit costs significantly. In the medical industry, injection molding offers the advantage of precise, repeatable production of small, complex parts that are often required for devices like syringes, catheters, and diagnostic tools. As a result, injection molding tends to be more cost-effective for large-scale production of small and intricate medical components, where the cost per part becomes much lower with higher volumes.
What is the waste of injection molding?
Injection molding is an efficient process, but like any manufacturing technique, it does produce some waste, which needs to be carefully managed, especially in medical device manufacturing where contamination and quality control are crucial.
Scrap Material: During the injection molding process, waste can be generated in the form of excess material that is not used in the final part. This often occurs when the molding material overflows the cavity or during the ejection process when parts are trimmed. In the medical industry, such waste materials can sometimes be recycled and reused, depending on the type of plastic and the cleanliness requirements of the medical parts.
Runner and Sprue Waste: These are the channels through which the molten plastic flows into the mold cavity. The material in the runners and sprues is often discarded after each injection cycle, as it does not form part of the final product. This waste is a significant contributor to material loss, but it can be recycled in many cases.
Defective Parts: Another form of waste comes from defective parts that fail to meet quality standards. For medical devices, this might include issues like dimensional inaccuracies or material defects, which could compromise the performance of the device. These defective parts are discarded, contributing to material waste.
Energy and Resource Use: While injection molding itself is energy-efficient, the resources used in the heating, cooling, and injection processes contribute to environmental impact. In the medical field, reducing energy consumption and optimizing the manufacturing process to minimize waste is an important part of sustainable production.
Managing waste effectively in injection molding involves careful process control, material recycling, and efficient mold design to minimize excess material and defective parts. In the medical industry, maintaining quality while reducing waste is essential for both cost control and compliance with regulatory standards.
What is the anatomy of the injection molding process?
The anatomy of the injection molding process refers to the various steps, components, and systems involved in manufacturing a plastic part. In the medical industry, this process is critical for producing high-quality, safe, and biocompatible components. Here’s a breakdown of the key elements of the process:
Injection Molding Machine: The injection molding machine is the primary equipment used in the process. It consists of a hopper for feeding plastic pellets, a heating chamber for melting the material, a screw or plunger to inject the molten plastic, and a mold clamping system.
Material Preparation: The raw material, typically plastic in the form of pellets, is loaded into the hopper. For medical products, the material needs to meet specific biocompatibility standards and may require sterilization before use.
Mold: The mold is the cavity or shape into which the molten plastic is injected. It is usually made of high-strength steel or aluminum and is precisely designed to create the desired part. In the medical industry, the mold design is critical to ensure that the final part meets strict dimensional tolerances and functionality requirements.
Injection and Cooling: Once the material is melted, it is injected into the mold under high pressure. After filling the mold, the material is allowed to cool, during which it solidifies into the desired shape.
Ejection: After the part has cooled and solidified, the mold opens, and the finished part is ejected from the mold. Automated systems are typically used for this stage to maintain speed and precision, especially in medical device production.
Post-processing: After the part is ejected, it may require further processing, such as trimming excess material, cleaning, or sterilization, depending on its intended medical use. Quality checks are conducted to ensure the part meets safety standards.
Each of these steps plays a crucial role in producing precise and reliable medical components through injection molding, ensuring that the parts are safe, durable, and compliant with regulatory requirements.
What is the medical term moulding mean?
In medical terminology, “moulding” refers to the shaping or forming of a body part, often in the context of molding a cast or a prosthesis. For example, in orthopedics, the term “moulding” can describe the process of shaping a material, such as plaster or thermoplastic, to conform to a part of the body, like an arm or leg, for the purpose of immobilization or support.
Moulding is a critical process in the medical field, especially in the creation of custom-made devices like orthopedic casts, braces, and prosthetic limbs. The goal is to create a device that fits the patient’s unique anatomy, ensuring comfort, function, and support. In this context, moulding involves using materials that can be molded into a specific shape and hardened or cooled to maintain that shape, providing the necessary support or correction.
In the context of injection molding in the medical field, the term can also refer to the process of forming medical components, such as dental implants or surgical tools, by shaping plastic or metal materials into precise forms that meet patient needs.
What is the automation molding process?
Automation molding refers to the use of automated systems to perform the various tasks in the injection molding process. This can include automated material feeding, molding, cooling, ejection, and post-processing. In medical manufacturing, automation is key to ensuring precision, reducing human error, and increasing the speed and efficiency of production. Automated systems often include robotics for part ejection and material handling, reducing labor costs and ensuring consistency across large volumes of medical products, such as syringes, diagnostic equipment parts, or surgical instruments.
Automated molding systems are also equipped with sensors and control systems to monitor and adjust key parameters like temperature, pressure, and cycle time in real time. In medical applications, this is crucial for maintaining strict quality standards and ensuring that each part meets the necessary specifications for biocompatibility, safety, and performance. Automation is essential in producing medical components with high precision, reliability, and at scale, which is important for applications where product consistency can directly impact patient safety.
What type of process is injection molding?
Injection molding is a type of manufacturing process used to create plastic parts and components, typically through a thermoplastic material that is injected into a mold cavity under high pressure. The process falls under the category of thermoplastic molding and is widely used in the production of medical devices, such as disposable syringes, catheters, and surgical tools. It is classified as a high-volume, precision manufacturing process, ideal for producing complex, intricate parts with consistent quality.
The key characteristic of injection molding is its ability to produce parts with tight tolerances and high repeatability, which is crucial for medical applications where precision is vital. Injection molding is highly adaptable, as it can handle a wide range of materials, from simple plastics to more advanced biocompatible materials, ensuring that it remains relevant for various medical applications.
How many types of molding processes are there?
There are several types of molding processes used in manufacturing, each suited for different applications. In the context of medical manufacturing, the most common types include:
Injection Molding: The most widely used method, ideal for producing precise, high-volume parts, such as surgical tools and diagnostic equipment components.
Blow Molding: Used to create hollow parts, such as bottles and medical containers.
Compression Molding: Suitable for thermosetting plastics, often used for medical components like rubber seals and gaskets.
Reaction Injection Molding (RIM): Utilized for producing large, lightweight parts, such as prosthetics and medical imaging equipment parts.
Rotational Molding: Used for creating hollow, large parts, often in the production of medical equipment cases or containers.
Thermoforming: Involves heating a plastic sheet and forming it over a mold, commonly used for packaging medical devices or creating trays for sterile products.
These processes each have distinct characteristics that make them suitable for specific medical applications, depending on the material properties, part complexity, and production volume required.
Is injection molding the same as injection compression molding?
Injection molding and injection compression molding are related but distinctly different processes. In standard injection molding, a thermoplastic material is heated until molten and then injected under high pressure into a fully closed mold cavity. The material fills the cavity completely and cools down to form the final part. This method is widely used in the medical industry for creating items like syringe barrels, housings for diagnostic devices, and other precision components where dimensional stability and repeatability are critical.
In contrast, injection compression molding is a hybrid process that combines aspects of both injection molding and compression molding. In this method, the mold is not entirely closed during the initial phase of material injection. Instead, the material is first injected into a partially open mold, allowing it to flow freely. Once the molten material is in place, the mold is further closed, applying compression that forces the material to fill every detail of the cavity. This additional compression reduces internal stresses, minimizes defects such as warpage or sink marks, and often results in superior surface finish and dimensional accuracy.
In medical applications, where even slight imperfections can affect device performance or patient safety, the injection compression molding process offers significant benefits. The enhanced uniformity of parts is especially valuable for producing components used in critical areas such as implantable devices or precision instruments for diagnostics. However, this process may require more sophisticated machinery and tighter process controls compared to standard injection molding. In summary, while both processes involve injecting material into a mold, injection compression molding’s unique two-step approach distinguishes it from conventional injection molding, offering improved part quality for sensitive medical applications.
What are the pros and cons of injection molding?
Injection molding is one of the most prevalent manufacturing processes, particularly in the medical industry, due to its high precision and ability to produce complex parts in large volumes. One of its primary advantages is the production efficiency—once a mold is created, thousands or even millions of identical parts can be produced with remarkable consistency. This repeatability is crucial for medical devices such as surgical instruments, diagnostic components, and single-use medical disposables, where precision and reliability directly impact patient safety and product performance.
Another significant advantage is the versatility of materials. Injection molding can work with a variety of biocompatible plastics, including polypropylene, polyethylene, and polycarbonate, ensuring that the end products meet stringent medical standards. The process also allows for complex geometries, fine details, and integrated features such as snap-fits or living hinges, all of which are valuable in medical device design. Additionally, the cycle time can be relatively short, leading to faster production rates and lower per-unit costs when high volumes are involved.
However, there are notable disadvantages as well. The initial investment for injection molding is high due to the cost of designing and fabricating precision molds. This upfront expense can be a barrier for low-volume or custom production runs. Moreover, once a mold is produced, making design changes can be difficult and expensive, which may limit flexibility during the development phase. The process also requires precise control of variables like temperature, pressure, and cooling times; any deviations can lead to defects such as warping, voids, or incomplete filling. In medical applications, these defects are particularly problematic as they could compromise device integrity and patient safety.
Balancing these pros and cons is essential. While the high setup cost and stringent process controls pose challenges, the benefits of high precision, repeatability, and scalability make injection molding a preferred method for many medical device manufacturers who must adhere to rigorous quality standards and regulatory requirements.
What is the difference between injection molding and LSR molding?
Injection molding and Liquid Silicone Rubber (LSR) molding are both processes used to create complex parts, yet they differ significantly in materials, processing conditions, and applications, particularly in the medical field. Traditional injection molding is primarily used with thermoplastics—materials that become pliable when heated and solidify upon cooling. This process involves melting plastic pellets and injecting the molten material into a mold, where it cools rapidly. It is widely used in producing high-precision components such as housings for medical devices, surgical instruments, and disposable medical products.
LSR molding, on the other hand, involves the use of liquid silicone rubber, a material known for its excellent biocompatibility, flexibility, and resistance to extreme temperatures. Unlike thermoplastics, LSR is not melted but remains in a liquid state until it is injected into a heated mold where a curing process, often catalyzed by a platinum-based catalyst, solidifies the material. This curing process allows for the creation of parts with very smooth surfaces, intricate details, and superior elasticity. In the medical arena, LSR molding is particularly valued for manufacturing soft-touch components, seals, gaskets, and other flexible parts that come in contact with the human body, such as in prosthetics, catheter components, and facial masks.
Another key difference is the processing temperature and pressures involved. LSR molding generally requires lower injection pressures compared to conventional thermoplastic injection molding and operates at a narrower processing window due to the curing reaction. While both processes require precise control, the chemistry and mechanics behind LSR molding add a layer of complexity that must be carefully managed to ensure consistency and performance. In summary, while both methods are valuable in medical device manufacturing, the choice between them depends largely on the material properties required and the specific application of the final product.
Is injection molding stronger than 3D printing?
When comparing injection molding and 3D printing, particularly for applications in the medical field, several factors come into play, including material properties, production consistency, and mechanical strength. Injection molding is renowned for producing parts with excellent mechanical strength, high durability, and consistent quality across high-volume production. This is because the process uses high-pressure injection of molten material into a precision-engineered mold, ensuring that the resulting parts have uniform density and mechanical properties. For medical devices like surgical instruments, implant housings, or diagnostic equipment components, this consistency is critical for reliability and patient safety.
3D printing, or additive manufacturing, offers tremendous flexibility for rapid prototyping and customization. It builds parts layer by layer from various materials, including polymers, resins, and even metals. While 3D printing is incredibly valuable for creating complex, custom geometries and for low-volume production runs, the mechanical strength of printed parts can vary. Many 3D printed parts, particularly those produced by fused deposition modeling (FDM), may have anisotropic properties—meaning the strength can differ depending on the direction of the layers. In contrast, injection molded parts typically have isotropic properties, making them uniformly strong in all directions.
Moreover, injection molding is optimized for high-volume production, leading to lower per-unit costs once the mold is fabricated. Although the initial setup for injection molding is expensive, the process yields parts that meet the rigorous standards required in the medical industry, such as those for biocompatibility and sterilization. In summary, while 3D printing excels in design flexibility and speed for prototyping, injection molding generally produces stronger, more consistent, and higher-quality parts that are better suited for the demanding applications found in medical device manufacturing.
What is the difference between CNC and injection molding?
CNC (Computer Numerical Control) machining and injection molding are two fundamentally different manufacturing processes, each with its unique advantages and applications in the medical field. CNC machining is a subtractive manufacturing process that involves removing material from a solid block (or billet) using computer-controlled tools. This method is ideal for producing parts that require high precision and tight tolerances, such as custom surgical instruments, implant components, or intricate diagnostic equipment housings. CNC machining allows for the creation of complex geometries and fine details and is highly flexible in terms of design modifications, making it valuable during the prototyping and low-volume production phases.
Injection molding, conversely, is an additive process where molten material is injected into a mold cavity under high pressure and then cooled to form a part. This process is exceptionally well-suited for high-volume production of components with consistent quality and repeatability. In the medical industry, injection molding is commonly used to produce disposable items such as syringes, catheters, and diagnostic device housings, where uniformity and biocompatibility are essential. The process is highly efficient once the initial mold is fabricated, although the upfront costs for mold design and tooling can be substantial.
The key differences lie in production volume, cost efficiency, and material utilization. CNC machining is generally more expensive per part for high-volume production because it is a slower, more labor-intensive process. However, it offers unmatched precision and is highly adaptable for custom or low-run manufacturing. Injection molding, on the other hand, offers rapid cycle times and low per-unit costs once the mold is made, making it the preferred choice for large-scale manufacturing.
In summary, while both CNC machining and injection molding are critical in the production of medical devices, the selection between them depends on the required volume, design complexity, material properties, and budget constraints. Each process serves different needs within the medical manufacturing ecosystem, ensuring that devices meet the strict quality and performance standards necessary for patient safety.
Contact our professional team now for a same-day quote and risk-free project evaluation. Benefit from ISO 8 cleanrooms, advanced machines, and 12-hour fast response in medical injection molding.